Humans are each unique in their biological makeup, and that presents a significant challenge for scientists studying how the environment affects health and well-being. For example, one individual’s exposure to a toxicant may increase his or her chance of experiencing an adverse health effect, and in another person, there may be little or no cause for concern. Research models sometimes fail to account for such population diversity, which limits understanding of complex gene-environment interactions as well as the ability to apply new findings in clinical settings.
Fernando Pardo Manuel de Villena, Ph.D., chair of the genetics department at the University of North Carolina at Chapel Hill, recently spoke with me about his work to incorporate greater genetic variability into his study designs, thereby increasing their human relevance. An NIEHS grant recipient, Pardo Manuel de Villena has been a global leader in related efforts for more than two decades. I spoke with him about his latest research, how environmental health scientists can better integrate insights from genetics, and his early intellectual influences (see sidebar).
Focused on human relevance
Rick Woychik: Can you talk about the history behind efforts by you and other scientists from around the world to incorporate greater genetic diversity into toxicological research?
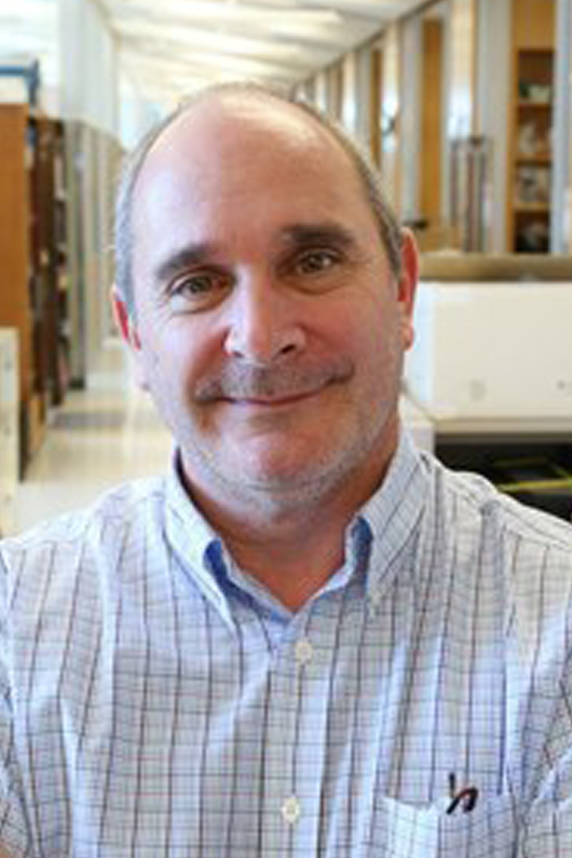
Fernando Pardo Manuel de Villena: In 2001, we were in Edinburgh, Scotland, for the International Mammalian Genome Society meeting, along with about 500 other researchers — a huge gathering. We decided we needed to move beyond the 20th century idea of genetics, where you look at one gene at a time and make generalizations, or else the human geneticists were going to leave us in the dust. Remember, this was around the time of the first-ever sequencing of the human genome, and we knew that such advancement would be revolutionary.
So, we thought about how to create mammalian populations that incorporate enough genetic variation that they become relevant not for one disease but for every biomedical trait. In other words, instead of selecting for one health effect or condition, we decided to think broadly and develop an approach that would be helpful for research involving toxicology, cancer, mental health, and so forth. Those conversations set in motion important work to integrate biological data across a variety of diseases and timepoints and to build the necessary research tools.
We understood that a single inbred mouse strain is not a model of human disease. It is impossible to make generalizations about whatever it is you are studying, such as the effects of a drug or an environmental exposure. You can get data, but those data are not broadly applicable — you don’t know whether they are important for the mean of the population, or the extreme, and inbred strains tend to be the extremes by the nature of their genetics.
That thinking eventually led to the development of the diversity outbred and collaborative cross models. The former enables very precise genetic mapping, which helps researchers better understand complex genetic traits and how they correlate with a phenotype, or an observable characteristic. The latter builds on 100 years of mouse genetics, creating a population-based model where genomes are reproducible and almost all genetic variation is captured, which helps scientists pinpoint specific genes associated with a certain health effect.
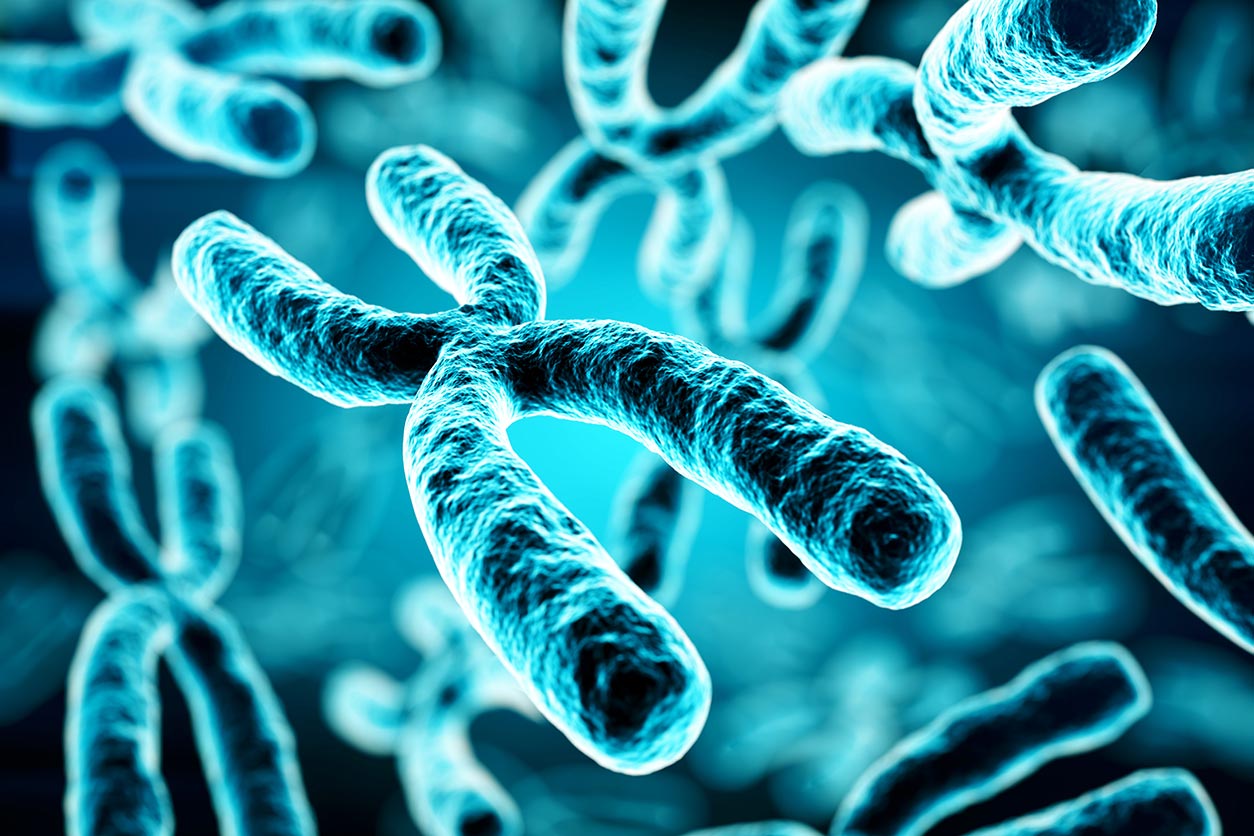
Arsenic exposure, complex gene interactions
RW: Yes, a single inbred mouse strain is very artificial — it is ideal for doing experiments, but it is not a truly viable way to model complex human disease, which is why it is great that the approaches you mentioned are now available to the scientific community. Can you talk a little bit about your current research?
FPMdV: Sure. So, right now, we are looking at the consequences of arsenic exposure — specifically, the interaction between genetic variation, arsenic exposure, nutrition, obesity, and type 2 diabetes. We want to expand what has been a fairly limited way to study arsenic, which is a very important toxicant, affecting hundreds of millions of people around the world.
There are fundamental physiological differences between mice and humans, and if we are going to try to figure out the complex interaction between the environment and genetics, we need to make sure that we do so in a way that is relevant to humans.
In the context of arsenic, the reason mice are not relevant to humans is that mice metabolize arsenic exceedingly well, which is not the case with people. So, our collaborators developed one mouse line in which they replaced the relevant mouse gene with the functionally equivalent human gene, and they did it in a very elegant way. The mice now metabolize arsenic in a way that is similar to humans, which is great because we have now combined that mouse strain in our collection of collaborative cross lines, and we are beginning to see very exciting things.
We have a relevant model, and we have genetic diversity. Now we can start to dig deeper and uncover the complex traits involved in response to arsenic exposure. After exposure, the substance is everywhere in the organism, and we don’t know whether the detoxification rate is different in the liver versus the bladder, the kidney, and so forth. With our current study design, we can address those questions, and that is why this project is so exciting to me.
Education, new partnerships key
RW: Looking at the broad-based environmental health sciences community, what knowledge gaps or issues do you think need to be addressed so that researchers can begin to incorporate greater genetic diversity in their studies and increase the human relevance of their work?
FPMdV: One area that will be especially important going forward is our data science infrastructure. Information generated through the kind of research you are talking about is not something that we can do in an Excel spreadsheet — that’s just not going to work. Developing and maintaining a robust infrastructure is absolutely key.
Education also is important. Geneticists need an education in toxicology and environmental health sciences, and toxicologists and environmental health scientists need an education in complex genetics. This is not about “who is right” but about working together to solve complex problems.
Take the collaborative cross model as an example. It is fundamentally based on integration, which in turn is based on the number of users and the number of phenotypes and doing rigorous science. If only a small community is doing that kind of research, it is not going to be powerful; what we need are scientists from different disciplines coming together to identify gaps and make our models even better.
(Rick Woychik, Ph.D., directs NIEHS and the National Toxicology Program.)
Source link
factor.niehs.nih.gov